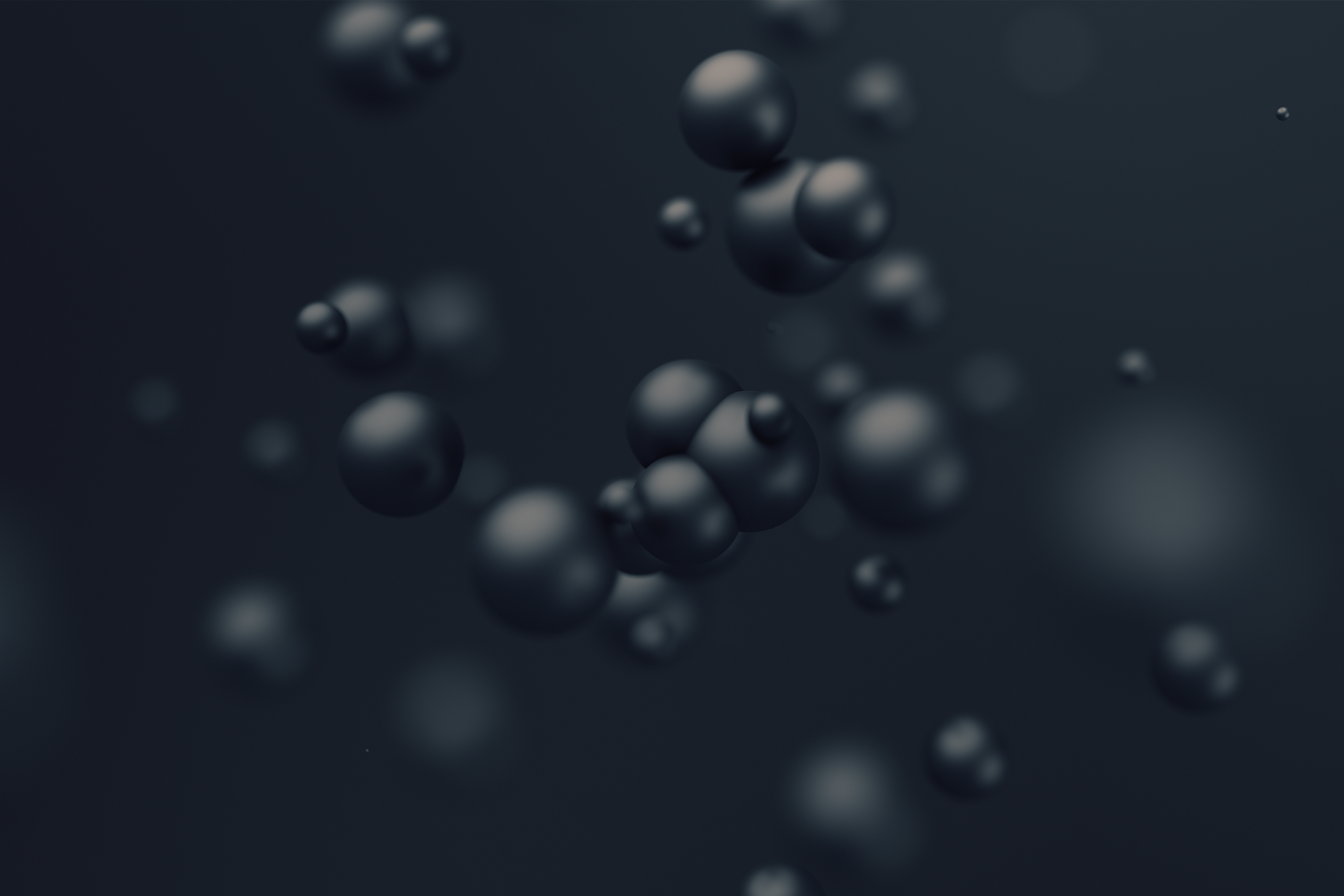
Blog
Share blog with everyone.
Recent Blogs
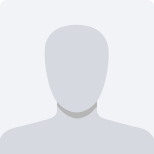
Phd Scholar Namrata Gaikwad, Prof Jitendra Sangwai and Prof Rajnish Kumar, IIT Madras, Prof Praveen Linga, National University of Singapore
Separation of Coal Mine Methane Gas mixture via sII and sH hydrate formation
Coal mine methane (CMM) also known as coal bed methane (CBM) is an unconventional natural gas resource and a significant source of clean energy, which is produced and emitted continuously from coal mine activities either from the coal seam itself or from other gassy formations available underground, mainly consisting of methane (CH4) and air [1], [2]. The exploitation of CMM is done mostly during the extraction of coal through underground mining. The release of CMM gas into the atmosphere is a wastage of natural gas resources, while it also contributes to global warming, as the greenhouse index of CH4 is 21 times higher than CO2. The separation of methane from CMM gas is thus desirable from an economic as well as an environmental point of view. A mature process such as methane capture and utilization from CMM gas would serve as an additional source of energy and provides a safer coal mine operation. The separation of methane before releasing it into the atmosphere would mitigate the global warming problem by reducing greenhouse gas emissions to the atmosphere. The operating cost of conventional gas separation methods is not in tune with the market requirements of being economical and safe processes. Thus, low cost, safe, efficient, and sustainable separation method for methane from its associated gases in CMM is still desirable. The hydrate-based gas separation process is a promising solution for CMM gas separation as it requires benign chemicals in low concentration, leading to low inventory and recycle cost, whereas the conventional separation methods require chemicals which may not be necessarily benign. Further, if the process is optimized properly, it may produce enriched methane as the main product and contaminant free water as a by-product from the same stream and requires much lesser energy during regeneration of the working liquid In their work, authors have considered CMM as a mixture of methane and nitrogen (CH4-N2, 30:70 mol%), and this gas mixture has been separated by the hydrate-based gas separation (HBGS) process. Formation of sI hydrate with 70% N2 in the mixture requires significantly higher pressure and thus not suitable for scale-up. Therefore, in this work with a suitable thermodynamic promoter, sII and sH hydrates were formed to study the methane separation from CMM gas mixture at moderate temperature and pressure conditions. sII hydrate formation was carried out using polar THF (Tetrahydrofuran) and non-polar cyclopentane (CP) at different molar concentrations and at 274.2 K temperature. sH hydrate formation was facilitated using polar tert-butyl-methyl-ether (TBME) and non-polar neo-hexane (NH) at different molar concentrations and at 274.2 K temperature. Further, water-soluble hydrate promoter sodium dodecyl sulfate (SDS) was used at 500 ppm to enhance the rate of hydrate formation and thus to achieve better separation efficiency in a given time. For the CMM gas mixture, sII hydrate formation showed better methane recovery compared to sH hydrate formation, whereas sH hydrate formation showed a better separation factor compared to sII hydrate formation. Hydrate dissociation was also carried out to recover the hydrated gas via depressurization and thermal stimulation to compare the effect of polar and non-polar hydrate formers. The authors have explored the gas hydrate-based method and based upon the experimental findings, an economical process may be developed for the separation of the gases from the mixture of nitrogen and methane. The work also provides insights about the economically feasible way for the storage of the methane under controlled conditions, requiring near to water freezing temperatures.
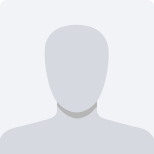
Ajay Koushik V
Carbon dioxide isn’t toxic! Listen to Nature!
We can learn many things by observing nature. She is the best teacher, so why not go back and ask her to hint about how to fix a serious issue that we are all concerned about right now- Carbon dioxide. Coal, Oil, and Gas- A Historical Outlook All biological systems like humans, animals, plants, and microbes are primarily composed of carbon, oxygen, and hydrogen. These creatures, when buried, experienced high pressure and temperature conditions under the soil for millions of years. Based on the location and the chemical composition of these fossils, eventually, they got converted into either solid, liquid, or gas. These solids are what we find as coal now. The liquids and gases are crude oil and natural gas, respectively. These are the primary fuels that we use in various vital applications such as manufacturing and transport and are known as fossil fuels. Apart from playing a crucial role in supplying energy, they directly or indirectly dictate how politics and policies play around various nations (but this is a story for later). What is a Fuel, and why does it cause CO2 emissions? In rough words, fuel is any substance that can emit energy when reacted with other substances. The energy stored in fuels as chemical bonds gets released while combusting them. This energy can be used in the applications described above. Typically, we burn the fuel in the presence of oxygen, and this ends up producing by-products along with energy. Since all these fuels are built by carbon primarily, its reaction with oxygen produces CO2 as the primary by-product. Other emission products, such as CO and NOx, are out of the scope of this article. Wasn’t there any CO2 before we started to exploit fossil fuels? CO2 has always been a part of ambient air. Decomposition, respiration, and ocean release are the “natural” sources of CO2. Then how were the CO2 levels kept in control for centuries? The answer is photosynthesis. It is critical to note that CO2 is not a toxic gas, and it is a vital part of the carbon cycle that facilitates life on this planet. In other words, without CO2, life wouldn’t be possible on earth. The plants/trees on this planet were sufficient to capture the excess ambient CO2 and water and convert them into starch and oxygen with the help of sunlight. However, now we have more CO2 (thanks to the increased usage of fossil fuels) and fewer plants/trees than before that could capture and utilize the CO2 in photosynthesis, leading to the accumulation of CO2 in the atmosphere. What does photosynthesis teach us? In photosynthesis, plants capture CO2 and upgrade it to starch (a useful chemical) using water (the source of hydrogen) and energy. In short, CO2 is used to produce a valuable substance. What if we try to emulate this process in the laboratory? Most of the day-to-day products we use are made up of carbon. Why not use the excess flue CO2 produced to produce such products? Almost every fuel we use is made up of carbon. We can also produce fuels from CO2 and use them as an energy carrier for various transport and industrial applications. Take a look at the below equations (this is not a typical scary textbook chemical equation). Carbon dioxide + Water → Starch (Natural process (Photosynthesis)) Carbon dioxide + Hydrogen (which comes from water) → Useful products (Artificial process) The simplified chemical equations clearly emphasize the lesson nature gives us on utilizing and managing CO2. The useful product could be anything ranging from methane, methanol, CO, syngas, dimethyl ether, formic acid, formaldehyde, higher hydrocarbons, aromatics, plastics, and so on, depending on the catalyst and the reaction conditions. For instance, Carbon Recycling International (CRI) is an Iceland-based company that produces methanol from CO2 captured from a coal-fired power plant. While the power plant provides the country with electricity, the methanol produced from the power plant’s emissions (they call it Vulcanol) is used as a fuel in automobiles, closing the cycle1. This process of capturing and utilizing CO2 is an attractive research area and an integral part of our CCUS (Carbon Capture Utilization and Storage) laboratory. What are the significant challenges involved in achieving this? Any chemical reaction will have two significant challenges- sourcing raw materials and ‘selectivity’ towards the desired products. The two raw materials we require for this process are carbon dioxide and hydrogen. Although CO2 is found in excess (which is why we are trying to reduce it), it is challenging to capture CO2 from various industrial and automobile sources and separate them, as they are usually found with other impurities such as sulfur and nitrogen oxides. Different separation techniques such as adsorption, absorption, membrane separation, and cryogenic separation are researched to separate CO2 from this mixture. It is also worth noting that CO2 is a highly stable molecule, making it difficult to undergo chemical changes. Hence various new catalysts and novel reactor configurations are being developed to make CO2 react to form valuable products. The next major challenge is the origin of hydrogen. Hydrogen is categorized with various colors based on its source (details to follow in the upcoming articles). In short, if hydrogen is obtained from a carbonaceous source such as coal, it is usually given the color grey/brown, meaning that they are not very environment-friendly as they are accompanied by the emission of CO2 and other greenhouse gases. However, if we could produce hydrogen from water splitting (as we learned from photosynthesis) using renewable energy, it would be called “Green Hydrogen”. Although this process is environmentally friendly, the economics of green hydrogen production would determine the feasibility of this process. Hence, optimization of water electrolysis (the process of separating hydrogen from water) becomes a significant research area. A few reaction pathways use compounds such as ammonia (NH3) and methane (CH4) as the source of hydrogen. There are also strategies where electrochemical or photochemical or a combination of both can generate hydrogen. We will discuss these approaches in detail in our upcoming articles. Additionally, there is a challenge in obtaining selectivity. Since carbon dioxide and hydrogen could react to produce many different products, it is essential to deduce the suitable catalyst and define the reaction conditions at which a particular product would form. For instance, CO2 and hydrogen produce methane (a gas) on nickel-based catalysts at atmospheric pressure, whereas methanol (a liquid) on copper-based catalysts at elevated pressures. Hence, understanding these reaction systems is crucial to designing appropriate catalysts and driving the reaction in the desired direction. These are a few among the diverse research directions we are working on in our CCUS laboratory. What is the future of such CO2 utilization projects? CO2 emission occurs predominantly from cement, iron and steel, and coal-fired power plants. These sectors contribute significantly to a country’s economy and couldn’t be overlooked. Hence, we can source vast amounts of CO2 from such industries for producing valuable chemicals. We can also think of integrating CO2 utilization plants with these emitters so that they can have the option of making useful chemicals/fuels from the waste CO2 they generate. If we synthesize fuels such as methane and methanol from waste CO2, they can be used back as fuel in the same industry, reducing their dependency on coal (also reducing its pulverizing cost). Lesser coal would correspond to lesser mining costs and easier waste handling, as liquid and gaseous fuels don’t produce ash, unlike coal. Commercialization of such fuels in the manufacturing and transport sectors would also enable us to minimize foreign dependence on oil, placing us much better economically.
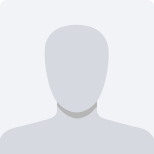
Nathan Sathish Kumar
Methanol Economy- A Road that leads to the Hydrogen Economy.
Several advantages and challenges in using hydrogen as fuel were addressed in our previous article. There is no doubt in hydrogen’s ability to fill the shoes of a clean and efficient fuel that we expect. However, due to technological, sourcing, political, policy-making, and safety reasons, it might take a while before hydrogen could replace the existing fossil fuels. At the same time, we can’t continue the usage of fossil fuels at the rate we are using now due to several environmental reasons. To bridge this gap, where we reduce the usage of fossil fuels and start using hydrogen as a fuel, we need to find ways to store and transport energy efficiently. Methanol (CH3OH) is a liquid at room temperature and is the simplest of alcohols that would enable us to bridge this gap. It not only boils at relatively lower temperatures (~65°C), but it also has the highest hydrogen to carbon ratio for any liquid fuel at room temperature, making it a cleaner fuel. This article discusses various aspects of the “Methanol Economy” and how crucial it is in tackling the modern-day energy-economics-environment war. Methanol has multiple applications. Methanol is a commodity chemical and is used as a feedstock for producing various other chemicals such as formaldehyde and olefins. These can be used to synthesize chemical products such as polymers that touch our day-to-day lives. Currently, these products are manufactured from fossil sources. Moreover, in its pure form, methanol can be used as a fuel in internal combustion engines or by mixing with gasoline. Nowadays, methanol is seen as a promising marine fuel by the major players in the ship industries, such as Maersk and MAN. Maersk was recently in the news for ordering 12 carbon-neutral container vessels that would operate on methanol. This is because methanol as a fuel produces significantly lesser amounts of SOx, NOx, and particulate matter than conventional fuels. Methanol can also be used as cooking fuel. The Government of Assam conducted the Methanol Cooking Fuel Program in 2018, creating awareness about using methanol as a cooking fuel that eventually would enhance indoor air quality. Furthermore, since a single methanol molecule can carry four hydrogen atoms, it can be used as a hydrogen carrier. Being a liquid, the existing infrastructures can easily be leveraged to store and transport methanol. Renewable methanol is produced using the CO2 emitted from the flue gas and green hydrogen. This is looked into as a way to reduce the atmospheric CO2 concentrations (or at least as a way to mitigate its deleterious impacts) and to store the excess renewable energy. As discussed in the previous article, storage and transportation are the most difficult challenges in adapting hydrogen as a fuel. Renewable methanol can overcome these limitations until technological advancements occur in hydrogen storage and transport. Power to liquid (PtL) technologies such as methanol production from renewable resources help reduce intermittency in renewable power generation. Methanol can be “polygenerated”. It means that it can be produced from various sources such as syngas, CO2, biomass, and municipal solid waste and can effectively help in waste management. India is one of the largest coal reserves in the world. However, there are many environmental issues with using coal as a fuel. Instead, coal can be gasified to produce syngas, which can then be converted into methanol. Coal to fuels is seen as a viable option to utilize coal cleanly. Recently BHEL commissioned a pilot plant to produce methanol from coal. Since methanol can be made from various feedstocks, it can be locally produced, increasing the energy security of countries and reducing the foreign dependence on fuel. Awareness of renewable methanol is observed all along the globe. Iceland-based Carbon Recycling International (CRI) commissioned a 4000 tons renewable methanol production plant per year named after Noble Laurate Prof. George Olah. Further, they established a commercial-scale renewable methanol plant of 110000 tons per year in the Henan province, China. According to their web data, this plant recycles about 160000 tons of CO2 annually. In a nutshell, the “Methanol Economy” comprises three different functions for renewable methanol i. Methanol as a clean fuel ii. Methanol as energy storage and transport medium iii. Methanol as a feedstock for producing other chemicals Provided its advantages, Methanol Institute (MI) recommended several policies for adopting the methanol economy into the Indian energy and fuel mix. Some of the key recommendations are as follows, · Acknowledging methanol and hydrogen as key energy carriers · Facilitating R&D and pilot projects toward enhanced methanol production · Better supply chain network for methanol · Awareness and supportive policy landscape for methanol Researchers, corporates, government bodies, and start-ups worldwide are looking for an affordable alternative energy source that doesn’t harm the environment. In our first article, we discussed why we might not be able to completely move away from carbon-based energy sources in the near future. In our previous article, we discussed how hydrogen could be the answer to all these questions, but they had traces of carbon involved while producing them, making hydrogen (except green) “not so clean”. Also, hydrogen had several technical drawbacks in handling, storage, and transport. As we technologically move towards a hydrogen-based economy, reducing the dependence on fossil fuels is critical for several environmental and political reasons. Still, it has to be done mainly for the sake of the environment. Methanol could act as a bridge facilitating a smooth transition between the current generation fossil fuels to the next generation hydrogen fuels.
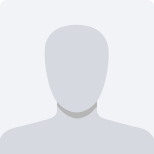
Murthi VK
H2, The Saviour?
Hydrogen was probably the first chemical we learned in our middle school chemistry lectures. It was taught as the smallest and simplest of elements. Its simple structure had nothing more than an electron and a proton, making it a student-friendly chemical element. Nevertheless, little did we know that the element we had all taken for granted during those days would be a game-changer in mitigating a serious universal concern- Global Warming. Our last article discussed nature’s way of handling emitted carbon dioxide. In this article, we will discuss the usage of different techniques to slow down the rate of consumption of fossil fuels. Climate change is horrifying, and we are already experiencing its flavour. The world is warming up, and immediate action has to be taken to mitigate this increase. On the other hand, the energy demand is expected to rise steeply given the rising population and increasing human needs (this statement might kick off a philosophical debate). As we all know, fossil fuels are the major energy suppliers even today. Most of the power, cement, and steel plants depend on coal as their energy source. Automobiles and household applications are fuelled by fossil oil and natural gas. These fossil fuels are primarily based on carbon and hydrogen, so that they might produce enormous amounts of carbon dioxide. Carbon dioxide is the most important greenhouse gas that contributes to global warming. Due to its uncertainty, a complete replacement of carbon-based fuels by renewable sources is highly irresolute, at least in the near future. In short, the circumstance is as follows: More needs drive increased fuel usage accompanied by increased emissions. On the other hand, our demands are still growing, giving us no scope to decrease the fuel usage. In this state of crisis, you might be thinking, “How good it would have been if there were a fuel that provides us with enormous energy without emissions?”-we heard you. And your prayers are answered!- There is a superhero fuel that burns without any emission but provides much energy to all our applications. Apart from all that, this fuel is abundant, making it commercially viable. It is said that complex problems have simple solutions. Indeed, the solution to this problem is a simple molecule, HYDROGEN. Why is hydrogen the key? Fuel is a chemical substance with energy “packed” as chemical bonds. We supply external heat to break those bonds, releasing those packets of energy. When we do this in a carbon-based fuel, it emits carbon dioxide. However, hydrogen does not contain any carbon, so that no carbon dioxide would be produced during this combustion. Water (or steam) would be the only by-product of hydrogen combustion, making it clean fuel. Unlike coal, burning hydrogen would not also result in ash formation. Additionally, hydrogen is packed with a lot more energy (~120 MJ/kg) than natural gas (~50 MJ/kg) and other fossil fuels, making it a robust contestant in the energy race. In short, hydrogen can produce electricity, fuel cars, and burn stoves without any emissions. What are the challenges in the way of using hydrogen as a fuel? We wouldn’t have overlooked something as significant as hydrogen for so many years if it had no limitations. Although hydrogen is clean and efficient fuel, its lightness, small size, and higher specific volume challenge its usage worldwide. Since hydrogen is light and small, they tend to diffuse through most of the materials in which it is stored or transported. Due to the same reason (small size), they occupy much space, necessitating huge containers or being stored/transported at higher pressures. Also, the condensation temperatures are as low as -250â°C, ruling out keeping/transporting it as a liquid. Furthermore, hydrogen can readily ignite when they contact air and burn with (close to) invisible flames. So, substantial safety measures and practices would have to be adopted if we were to use hydrogen as fuel worldwide. In addition to the foretold challenges, more significant challenges will be discussed in the paragraphs below. Source of hydrogen- A determining factor for the future of clean energy Unlike carbon-based fossil fuels, hydrogen is not found in its free form. In other words, it is always found along with other compounds such as water, coal, and methane. The biggest challenge in using hydrogen as a fuel is identifying the apt source of hydrogen. The source of hydrogen would determine if the used hydrogen is clean or if it has some “black” history. There is a trade-off between the “cleanliness” of hydrogen and its cost. Today, hydrogen produced from carbon-based sources is relatively cheaper than clean ones. But intuitively, it makes very little sense to produce a clean fuel accompanied by emissions. Based on the origin of hydrogen, it is categorized as having different colours. Coal is primarily composed of carbon, hydrogen, nitrogen, oxygen, and sulfur. Coal can undergo gasification (not the same as coal combustion) to produce a mixture of gases from which hydrogen can be separated. Hydrogen generated from coal is called brown hydrogen and is accompanied by carbon dioxide emissions. Natural gas predominantly contains methane (CH4). Every methane molecule has four hydrogen atoms (as seen from its chemical formula), and they can be separated using a variety of processes. There are different processes, such as steam reforming of methane (SRM), dry reforming of methane (DRM), and partial oxidation of methane (POx), where we use different techniques to produce hydrogen from natural gas. Although some emissions also accompany this process, it is slightly cleaner than the coal route, and the hydrogen so produced is named grey hydrogen. Carbon sequestration is a collection of expertise to store excess carbon dioxide in any place other than the atmosphere. Typically, they are kept under ocean beds, under the soil, or can be used for enhanced oil recovery techniques (an exciting topic to be discussed in our upcoming articles). If the plant where either the brown or the grey hydrogen (discussed above) has the facility to sequester carbon dioxide emitted during the process, rather than releasing it into the atmosphere, the hydrogen would be considered cleaner than before. The hydrogen produced from a carbon-based source complemented by the sequestration of carbon dioxide is called blue hydrogen. Methane (CH4) can undergo pyrolysis (heating in the absence of air) to form coke and hydrogen. This is a newly explored pathway where coke can be used as a fuel in various industrial applications. This could also be a slight disadvantage as we are trying to avoid the usage of solid fuels as they generate ash as a combustion by-product. The hydrogen produced by this pathway is termed turquoise hydrogen. In The Mysterious Island, Cyrus Harding mentioned, “Water will be the coal of the future.” This book was published in 1875, and it was indeed a visionary statement. The world has around 70% covered by water (H2O), and water has hydrogen. We could produce significantly large quantities of hydrogen from water without any carbonaceous emissions. Also, burning hydrogen for energy produces water as the by-product. Although this looks like a great deal, there is a significant challenge in making hydrogen from water. Water is a very stable molecule, and we need enormous energy to break it. Investing large magnitudes of energy into creating a fuel that would provide us back the energy is commercially a terrible idea. However, recent advancements in water electrolysis are proving to be promising for hydrogen production and one of the crucial areas of research in our CCUS laboratory. When we use renewable sources such as solar and wind as the source of electricity to produce hydrogen from water, it is called green hydrogen. If we use nuclear sources of electricity for the same, it is called purple hydrogen. Any greenhouse emissions would not accompany green and purple hydrogen production. As said above, the cleanliness of hydrogen increases with an increase in cost, making green hydrogen the costliest among the available sources (refer to the figure). However, intense research is being done on improving the efficiency of the production of green hydrogen, and we can expect it to be affordable soon in the future. The below chart shows the price comparison of hydrogen from different sources.
Separation of Coal Mine Methane Gas mixture via sII and sH hydrate formation
Coal mine methane (CMM) also known as coal bed methane (CBM) is an unconventional natural gas resource and a significant source of clean energy, which is produced and emitted continuously from coal mine activities either from the coal seam itself or from other gassy formations available underground, mainly consisting of methane (CH4) and air [1], [2]. The exploitation of CMM is done mostly during the extraction of coal through underground mining. The release of CMM gas into the atmosphere is a wastage of natural gas resources, while it also contributes to global warming, as the greenhouse index of CH4 is 21 times higher than CO2. The separation of methane from CMM gas is thus desirable from an economic as well as an environmental point of view. A mature process such as methane capture and utilization from CMM gas would serve as an additional source of energy and provides a safer coal mine operation. The separation of methane before releasing it into the atmosphere would mitigate the global warming problem by reducing greenhouse gas emissions to the atmosphere. The operating cost of conventional gas separation methods is not in tune with the market requirements of being economical and safe processes. Thus, low cost, safe, efficient, and sustainable separation method for methane from its associated gases in CMM is still desirable. The hydrate-based gas separation process is a promising solution for CMM gas separation as it requires benign chemicals in low concentration, leading to low inventory and recycle cost, whereas the conventional separation methods require chemicals which may not be necessarily benign. Further, if the process is optimized properly, it may produce enriched methane as the main product and contaminant free water as a by-product from the same stream and requires much lesser energy during regeneration of the working liquid In their work, authors have considered CMM as a mixture of methane and nitrogen (CH4-N2, 30:70 mol%), and this gas mixture has been separated by the hydrate-based gas separation (HBGS) process. Formation of sI hydrate with 70% N2 in the mixture requires significantly higher pressure and thus not suitable for scale-up. Therefore, in this work with a suitable thermodynamic promoter, sII and sH hydrates were formed to study the methane separation from CMM gas mixture at moderate temperature and pressure conditions. sII hydrate formation was carried out using polar THF (Tetrahydrofuran) and non-polar cyclopentane (CP) at different molar concentrations and at 274.2 K temperature. sH hydrate formation was facilitated using polar tert-butyl-methyl-ether (TBME) and non-polar neo-hexane (NH) at different molar concentrations and at 274.2 K temperature. Further, water-soluble hydrate promoter sodium dodecyl sulfate (SDS) was used at 500 ppm to enhance the rate of hydrate formation and thus to achieve better separation efficiency in a given time. For the CMM gas mixture, sII hydrate formation showed better methane recovery compared to sH hydrate formation, whereas sH hydrate formation showed a better separation factor compared to sII hydrate formation. Hydrate dissociation was also carried out to recover the hydrated gas via depressurization and thermal stimulation to compare the effect of polar and non-polar hydrate formers. The authors have explored the gas hydrate-based method and based upon the experimental findings, an economical process may be developed for the separation of the gases from the mixture of nitrogen and methane. The work also provides insights about the economically feasible way for the storage of the methane under controlled conditions, requiring near to water freezing temperatures.
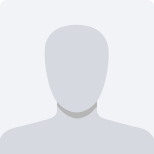
Phd Scholar Namrata Gaikwad, Prof Jitendra Sangwai and Prof Rajnish Kumar, IIT Madras, Prof Praveen Linga, National University of Singapore
Join our mailing list
Subscribe to our news letter and never miss our blogs, latest news,etc. Our news letter is sent once a week every monday